Unit 3 Equilibrium
3.2 Le Châtelier’s Principle
OpenStax
Section Learning Objectives
- Describe the ways in which an equilibrium system can be stressed.
- Predict the response of a stressed equilibrium using Le Châtelier’s principle.
✓ SECTION 3.2 CHECKLIST
Learning Activity | Graded? | Estimated Time |
---|---|---|
Make notes about this section’s reading portion. | No | 60 min |
Optional Resources: Watch suggested videos. | No | 7 min |
Optional Activities: Perform chemistry lab simulations. | No | 30 min |
Work on the self-check question. | No | 10 min |
Work on practice exercises. | No | 120 min |
📖 READING PORTION
A system at equilibrium is in a state of dynamic balance, with forward and reverse reactions taking place at equal rates. If an equilibrium system is subjected to a change in conditions (a stress) that affects these reaction rates differently, then the rates are no longer equal and the system is not at equilibrium. The system will subsequently experience a net reaction in the direction of greater rate (a shift) that will re-establish the equilibrium. This phenomenon is summarized by Le Châtelier’s principle: if an equilibrium system is stressed, the system will experience a shift in response to the stress that re-establishes equilibrium. Such stresses take the form of changes in concentration, and equivalently changes to partial pressure and volume for gaseous species, and temperature. We examine the effects of each stress.
Effect of a Change in Concentration
If an equilibrium system is subjected to a change in the concentration of a reactant or product species, the rate of either the forward or the reverse reaction will change. As an example, consider the equilibrium reaction
H2(g) + I2(g) ⇌ 2 HI(g)
The rate laws for the forward and reverse reactions are
forward H2(g) + I2(g) → 2 HI(g) ratef = kf[H2]m[I2]n
reverse 2 HI(g) → H2(g) + I2(g) rater = kr[HI]x
When this system is at equilibrium, the forward and reverse reaction rates are equal, ratef = rater. If the system is stressed by adding reactant, either H2 or I2, the resulting increase in concentration causes the rate of the forward reaction to increase, exceeding that of the reverse reaction, ratef > rater. The system will experience a temporary net reaction in the forward direction to re-establish equilibrium. The equilibrium will shift right, toward the products. This same rightward shift will result if some product HI is removed from the system, which decreases the rate of the reverse reaction, again resulting in the same imbalance in rates.
The same logic can be used to explain the left shift, toward the reactants that results from either removing reactant or adding product to an equilibrium system. These stresses both result in an increased rate for the reverse reaction, ratef < rater, and a temporary net reaction in the reverse direction to re-establish equilibrium.
Effect of a Change in Partial Pressure
For gas-phase equilibria such as H2(g) + I2(g) ⇌ 2 HI(g), the concentration of gaseous species is related to the partial pressure. Recall that the partial pressure p of an ideal gas is proportional to its molar concentration [ideal gas],
[latex][\text{ideal gas}] = \frac{n}{V} = \frac{p}{RT}[/latex]
and so changes in the partial pressures of any reactant or product are essentially changes in concentrations and thus yield the same effects on equilibria.
Effect of a Change in Reaction Volume for Gas Phase Reactions
Aside from adding or removing reactant or product, the pressures (concentrations) of species in a gas-phase equilibrium can also be changed by changing the volume occupied by the system. Since all species of a gas-phase equilibrium occupy the same volume, a given change in volume will cause the same change in concentration for both reactants and products. In order to discern what shift, if any, this type of stress will induce, the stoichiometry of the reaction must be considered.
Changes in volume does not affect the system H2(g) + I2(g) ⇌ 2 HI(g), but a different system,
2 NO2(g) ⇌ 2 NO(g) + O2(g)
however, yields a different result. In this case, an increase in the system’s total volume shifts the equilibrium to the right, toward the products. A decrease in volume shifts the equilibrium to the left, toward the reactant. These results illustrate the relationship between the stoichiometry of a gas phase equilibrium and the effect of a volume-induced pressure (concentration) change. If the total molar amounts of reactants and products are equal, as in the first example, a change in volume does not shift the equilibrium. If the molar amounts of reactants and products are different, a change in system volume will shift the equilibrium in a direction that better “accommodates” the volume change. In the second example, two moles of reactant (NO2) yield three moles of product (2 NO + O2), and so decreasing the system volume causes the equilibrium to shift left since the reverse reaction produces less gas (2 mol) than the forward reaction (3 mol). Conversely, increasing the volume of this equilibrium system would result in a shift towards products.
Moreover, any change in the volume of gases inversely affects the pressure. If pressure increases due to decrease in volume, as in this example, the reverse reaction will initially speed up more than the forward reaction since the reverse reaction involves three molecules (2 NO and 1 O2) whereas the forward reaction only involves two molecules (2 NO2). Thus, in this case, the equilibrium will shift towards the left. For similar reasons, an increase in volume has the opposite effect.
It should also be noted that not all reactants and products are in the gas state. A change in volume only affects reactants and products that are in the gas state. Solids and liquids are considered incompressible.
Effect of a Change in Temperature
Changes in concentration, partial pressure, and volume do not affect the relative amounts of products to reactants at equilibrium. However, temperature actually does change the position of equilibrium. Predicting the effect of temperature on an equilibrium is most conveniently accomplished by considering the enthalpy change of the reaction. For example, the decomposition of dinitrogen tetroxide is an endothermic (heat-consuming) process
N2O4(g) ⇌ 2 NO2(g) ΔH = +57.20 kJ
For purposes of applying Le Châtelier’s principle, heat (ΔH) may be viewed as a reactant
heat + N2O4(g) ⇌ 2 NO2(g), or
57.20 kJ + N2O4(g) ⇌ 2 NO2(g)
Raising the temperature of the system is akin to increasing the amount of a reactant but with the profound effect of moving the position of the equilibrium toward the right. The ratio of products to reactants will increase. There will be a net gain in the amount of products and a net loss in the amount of reactants. Lowering the system temperature will likewise cause the position of the equilibrium to move toward the left. The ratio of products to reactants will decrease. For exothermic processes, heat is viewed as a product of the reaction and so the opposite temperature dependence is observed.
An increase in temperature always initially speeds up endothermic reactions more than exothermic reactions. Conversely, a decrease in temperature always initially slows down endothermic reactions more than exothermic reactions. Recall that when the forward reaction is endothermic, the reverse reaction is exothermic. Thus, when temperature is increased, equilibrium always shifts towards the right (more products) when the forward reaction is endothermic. Likewise, a decrease in temperature will have the same effect when the forward reaction is exothermic. The opposite occurs for an increase in temperature when the forward reaction is exothermic or when temperature is decreased for an endothermic reaction.
Effect of a Catalyst
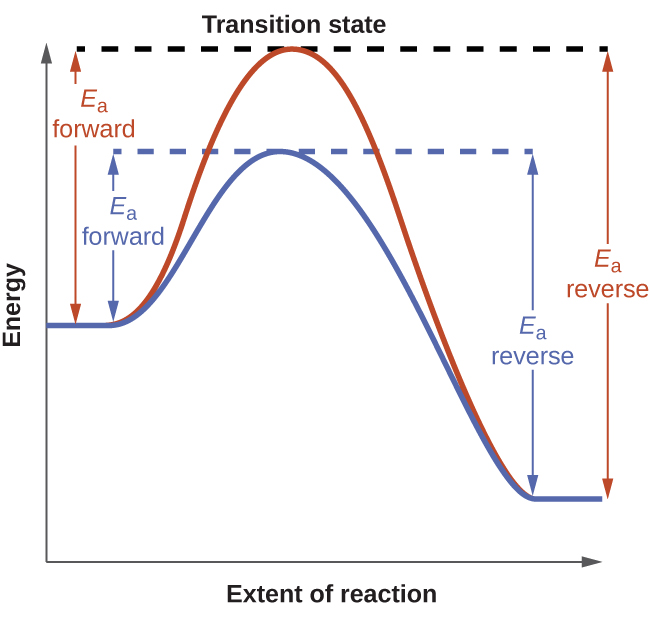
The kinetics unit identifies a catalyst as a substance that enables a reaction to proceed via a different mechanism with an accelerated rate. The catalyzed reaction mechanism involves a lower energy transition state than the uncatalyzed reaction, resulting in a lower activation energy, Ea, and a correspondingly greater rate constant.
To discern the effect of catalysis on an equilibrium system, consider the reaction diagram for a simple one-step (elementary) reaction shown in Figure 1. The lowered transition state energy of the catalyzed reaction results in lowered activation energies for both the forward and the reverse reactions. Consequently, both forward and reverse reactions are accelerated, and equilibrium is achieved more quickly but without a change in the relative amounts of reactants or products at equilibrium.
Example 1
Nickel reacts with carbon monoxide in the equation
Ni(s) + 4 CO(g) ⇌ Ni(CO)4(g)
How does increasing the CO partial pressure, decreasing the volume of the reaction, and adding nickel affect the equilibrium?
SOLUTION
Increasing the CO partial pressure. Increasing the reactant partial pressure shifts the equilibrium in favour of products.
Decreasing the volume of the reaction. There are more moles of gas in the reactant than the product side. Decreasing the volume shifts the equilibrium to the reactants.
Adding nickel. Adding or removing solid species does not affect the equilibrium as long as the solid species is present since pure solids (and pure liquids) have a constant concentration. A shift in equilibrium only occurs when there is a change in the concentrations of the aqueous solutes or gases involved in the reaction.
An interesting case study highlighting these equilibrium concepts is the industrial production of ammonia, NH3. This substance is among the “top 10” industrial chemicals with regard to production, with roughly two billion pounds produced annually in the US. Ammonia is used as a chemical feedstock to synthesize a wide range of commercially useful compounds, including fertilizers, plastics, dyes, and explosives. Most industrial production of ammonia uses the Haber-Bosch process based on the following equilibrium reaction
N2(g) + 3 H2(g) ⇌ 2 NH3(g) ΔH = −92.2 kJ
The traits of this reaction present challenges to its use in an efficient industrial process. There is little ammonia compared to reactants in the equilibrium mixture, and the rate of this reaction is relatively slow at low temperatures. To raise the yield of ammonia, the industrial process is designed to operate under conditions favoring product formation (Figure 2). High pressures (concentrations) of reactants are used, ~150−250 atm, to shift the equilibrium right, favoring product formation. Ammonia is continually removed (collected) from the equilibrium mixture during the process, lowering its concentration and also shifting the equilibrium right. Although low temperatures favor product formation for this exothermic process, the reaction rate at low temperatures is inefficiently slow. A catalyst is used to accelerate the reaction to reasonable rates at relatively moderate temperatures (400−500°C).
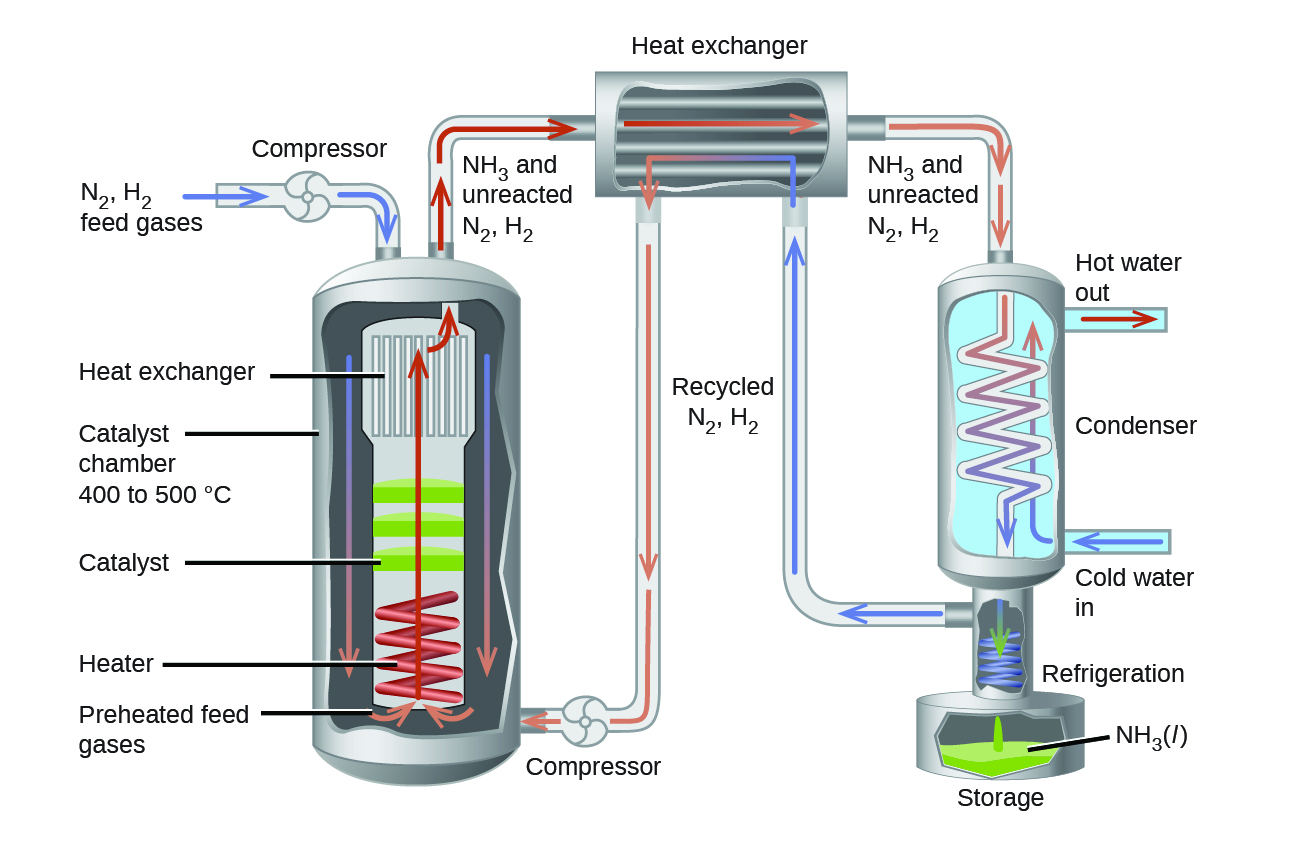
Optional Resources
Watch a video demonstration of the NO2 – N2O4 equilibrium.
Video 1 Volume Effect on Equilibrium – LeChatelier’s Principle Lab Extension (4
s).Watch a video about the Haber process.
Video 2 GCSE Chemistry – The Haber Process Explained (5 min 36 s).
Optional Activity
Perform a virtual lab of Le Châtelier’s principle – the effect of changing concentration, using the ChemReaX simulation app.
Perform a virtual lab of Le Châtelier’s principle – the effect of changing temperature, using the ChemReaX simulation app.
What will be the effect on the following equilibrium system if a catalyst is added?
Ca2+ (aq) + C2O42−(aq) ⇌ CaC2O4(s)
A) precipitation of more solid
B) an increase in the concentrations of Ca2+ and C2O42−
C) the equilibrium shifts toward the product
D) equilibrium is attained faster but does not affect the relative quantities of reactants and products at equilibrium
Click to see answer
D) equilibrium is attained faster but does not affect the relative quantities of reactants and products at equilibrium