Unit 6 Electrochemistry
6.2 Reduction Potentials
OpenStax
Section Learning Objectives
- Define reduction potential.
- Use a table of reduction potentials to predict whether a redox reaction can occur spontaneously.
- Describe how to construct an electrochemical cell.
✓ SECTION 6.2 CHECKLIST
Learning Activity | Graded? | Estimated Time |
---|---|---|
Make notes about this section’s reading portion. | No | 60 min |
Optional Activity: Perform simulated redox experiments. | No | 20 min |
Work on the self-check question. | No | 10 min |
Work on practice exercises. | No | 20 min |
📖 READING PORTION
Measuring Reduction Potentials
The reduction potential E, measured in volts, is the tendency of chemical species to accept electrons, i.e. be reduced. E° is the standard reduction potential, where the superscript “°” on the E denotes standard conditions (1 bar or 1 atm for gases, 1 M for solutes). Take for example the following reduction half-reactions and the corresponding standard reduction potentials. By convention, the standard reduction potential for hydrogen ion is set as the reference point zero. The reduction potentials of other species are measured relative to that of hydrogen ion.
[latex]\begin{align*} \text{Ag}^+(aq)\;+\;\text{e}^-\;&\longrightarrow\;\text{Ag}(s) &&E^{\circ}=+0.80\text{ V} \\ \text{Cu}^{2+}(aq)\;+\;2\text{e}^-\;&\longrightarrow\;\text{Cu}(s) &&E^{\circ}=+0.337\text{ V} \\ 2\text{H}^+(aq)\;+\;2\text{e}^-\;&\longrightarrow\;\text{H}_2(g) &&E^{\circ}=0\text{ V} \\ \text{Zn}^{2+}(aq)\;+\;2\text{e}^-\;&\longrightarrow\;\text{Zn}(s) &&E^{\circ}=-0.76\text{ V} \end{align*}[/latex]
We can use this arrangement to predict whether redox reactions will occur. Oxidizing agents are on the left side of the reduction half-reaction, and reducing agents are on the right. When two half-reactions are coupled together, the half-reaction with the higher reduction potential, meaning greater tendency to accept electrons, becomes the reduction half-reaction. The other half-reaction with the lower reduction potential, meaning lower tendency to accept electrons and thus greater tendency to be give electrons, is reversed and becomes the oxidation half-reaction. For a specific example between silver and zinc
[latex]\begin{align*} \text{Ag}^+(aq)\;+\;\text{e}^-\;&\longrightarrow\;\text{Ag}(s) &&E^{\circ}=+0.80\text{ V} \\ \text{Zn}^{2+}(aq)\;+\;2\text{e}^-\;&\longrightarrow\;\text{Zn}(s) &&E^{\circ}=-0.76\text{ V} \end{align*}[/latex]
The higher potential copper half-reaction stays as reduction. The lower potential zinc half-reaction is flipped into an oxidation half-reaction
[latex]\begin{align*} &\text{reduction:}&2\times\left\{\;\text{Ag}^{+}(aq)\;+\;\text{e}^{-}\;\right.&{\longrightarrow}\;\left.\text{Ag}(s)\right\} \\ &\text{oxidation:}&\;\text{Zn}(s) \;&{\longrightarrow}\;\text{Zn}^{2+}(aq)\;+\;2\text{e}^{-} \\ \hline &\text{overall:}&\;2\text{Ag}^{+}(aq)\;+\;\text{Zn}(s)\;&{\longrightarrow}\;2\text{Ag}(s)\;+\;\text{Zn}^{2+}(aq) \end{align*}[/latex]
Note that multiplying the entire half-reaction stoichiometry by a constant does not change the reduction potential of the half-reaction.
Based on the reduction potentials, we expect that
- Ag+ can oxidize Cu, H2, and Zn
- Cu2+ can oxidize H2 and Zn but not Ag
- Zn2+ cannot oxidize Ag, Cu, or H2
- Ag cannot reduce Cu2+, H+, or Zn2+
- H2 can reduce Ag+ and Cu2+ but not Zn2+
- Zn can reduce Ag+, Cu2+, and H2
and so on.
For redox reaction to occur spontaneously, there must be an oxidizing agent whose reduction half-reaction has higher (more positive) reduction potential and a reducing agent from a reduction half-reaction at a lower (more negative) reduction potential. In other words, the oxidizing agent must be located higher up on the list than the reducing agent. For example, the redox reaction between Cu as the reducing agent and Zn2+ as the oxidizing agent does not occur spontaneously because copper has a higher reduction potential than zinc.
The notion of more positive and more negative reduction potentials does not refer to the positive or negative value of E but rather the value of E in relation to each other. The standard reduction potential of the silver half-reaction is E° = +0.80 V and that of copper is E° = +0.337 V. The standard reduction potential of silver is more positive and that of copper is more negative even though both E° are more positive than the reference point, the H+ standard reduction potential.
The strongest oxidizing agent on the list of reduction potentials has the highest (most positive) E. Conversely, the strongest reducing agent is on the right side of the reduction half-reaction that has the lowest (most negative) E. On the list, the strongest oxidizing agent is Ag+ and the strongest reducing agent is Zn. Redox reactions occur between the strongest oxidizing agent and the strongest reducing agent. For example, by combining Cu2+ with H2 and Zn, the redox reaction will occur with Cu2+ as the oxidizing agent and Zn as the reducing agent. Zn is a stronger reducing agent than H2. Only after Zn is completely oxidized can Cu2+ oxidize H2.
No reaction is expected by combining two oxidizing agents, e.g. Ag+ and H+, or two reducing agents, e.g. Ag and H2.
Standard reduction potentials for selected reduction reactions are shown in Table 1. A more complete list is provided here (Source: OpenStax Chemistry 2e). The oxidizing agents and reducing agents may be one ion or molecule or a collection of ions and molecules. Using a table of half-reactions to balance redox reactions has the advantage over the method in Section 6.1 in that the half-reactions are already balanced. The reduction half-reaction stays as written on the table, and the half-reaction is flipped for the oxidation half-reaction. If needed, multiply the half-reaction stoichiometry by a constant to have the same number of electrons in the oxidation and reduction half-reactions.
Reduction Half-Reaction |
E° (V) |
[latex]\text{F}_2(g)\;+\;2\text{e}^{-}\;{\longrightarrow}\;2\text{F}^{-}(aq)[/latex] | +2.866 |
[latex]\text{PbO}_2(s)\;+\;\text{SO}_4^{\;\;2-}(aq)\;+\;4\text{H}^{+}(aq)\;+\;2\text{e}^{-}\;{\longrightarrow}\;\text{PbSO}_4(s)\;+\;2\text{H}_2\text{O}(l)[/latex] | +1.69 |
[latex]\text{MnO}_4^{\;\;-}(aq)\;+\;8\text{H}^{+}(aq)\;+\;5\text{e}^{-}\;{\longrightarrow}\;\text{Mn}^{2+}(aq)\;+\;4\text{H}_2\text{O}(l)[/latex] | +1.507 |
[latex]\text{Au}^{3+}(aq)\;+\;3\text{e}^{-}\;{\longrightarrow}\;\text{Au}(s)[/latex] | +1.498 |
[latex]\text{Cl}_2(g)\;+\;2\text{e}^{-}\;{\longrightarrow}\;2\text{Cl}^{-}(aq)[/latex] | +1.35827 |
[latex]\text{O}_2(g)\;+\;4\text{H}^{+}(aq)\;+\;4\text{e}^{-}\;{\longrightarrow}\;2\text{H}_2\text{O}(l)[/latex] | +1.229 |
[latex]\text{Pt}^{2+}(aq)\;+\;2\text{e}^{-}\;{\longrightarrow}\;\text{Pt}(s)[/latex] | +1.20 |
[latex]\text{Br}_2(aq)\;+\;2\text{e}^{-}\;{\longrightarrow}\;2\text{Br}^{-}(aq)[/latex] | +1.0873 |
[latex]\text{Ag}^{+}(aq)\;+\;\text{e}^{-}\;{\longrightarrow}\;\text{Ag}(s)[/latex] | +0.7996 |
[latex]\text{Hg}_2^{\;\;2+}(aq)\;+\;2\text{e}^{-}\;{\longrightarrow}\;2\text{Hg}(l)[/latex] | +0.7973 |
[latex]\text{Fe}^{3+}(aq)\;+\;\text{e}^{-}\;{\longrightarrow}\;\text{Fe}^{2+}(aq)[/latex] | +0.771 |
[latex]\text{MnO}_4^{\;\;-}(aq)\;+\;2\text{H}_2\text{O}(l)\;+\;3e^{-}\;{\longrightarrow}\;\text{MnO}_2(s)\;+\;4\text{OH}^{-}(aq)[/latex] | +0.558 |
[latex]\text{I}_2(s)\;+\;2\text{e}^{-}\;{\longrightarrow}\;2\text{I}^{-}(aq)[/latex] | +0.5355 |
[latex]\text{NiO}_2(s)\;+\;2\text{H}_2\text{O}(l)\;+\;2\text{e}^{-}\;{\longrightarrow}\;\text{Ni(OH)}_2(s)\;+\;2\text{OH}^{-}(aq)[/latex] | +0.49 |
[latex]\text{Cu}^{2+}(aq)\;+\;2\text{e}^{-}\;{\longrightarrow}\;\text{Cu}(s)[/latex] | +0.337 |
[latex]\text{Hg}_2\text{Cl}_2(s)\;+\;2\text{e}^{-}\;{\longrightarrow}\;2\text{Hg}(l)\;+\;2\text{Cl}^{-}(aq)[/latex] | +0.26808 |
[latex]\text{AgCl}(s)\;+\;\text{e}^{-}\;{\longrightarrow}\;\text{Ag}(s)\;+\;\text{Cl}^{-}(aq)[/latex] | +0.22233 |
[latex]\text{Sn}^{4+}(aq)\;+\;2\text{e}^{-}\;{\longrightarrow}\;\text{Sn}^{2+}(aq)[/latex] | +0.151 |
[latex]2\text{H}^{+}(aq)\;+\;2\text{e}^{-}\;{\longrightarrow}\;\text{H}_2(g)[/latex] | 0.00 |
[latex]\text{Pb}^{2+}(aq)\;+\;2\text{e}^{-}\;{\longrightarrow}\;\text{Pb}(s)[/latex] | −0.126 |
[latex]\text{Sn}^{2+}(aq)\;+\;2\text{e}^{-}\;{\longrightarrow}\;\text{Sn}(s)[/latex] | −0.1262 |
[latex]\text{Ni}^{2+}(aq)\;+\;2\text{e}^{-}\;{\longrightarrow}\;\text{Ni}(s)[/latex] | −0.257 |
[latex]\text{Co}^{2+}(aq)\;+\;2\text{e}^{-}\;{\longrightarrow}\;\text{Co}(s)[/latex] | −0.28 |
[latex]\text{PbSO}_4(s)\;+\;2\text{e}^{-}\;{\longrightarrow}\;\text{Pb}(s)\;+{\;\text{SO}_4}^{2-}(aq)[/latex] | −0.3505 |
[latex]\text{Cd}^{2+}(aq)\;+\;2\text{e}^{-}\;{\longrightarrow}\;\text{Cd}(s)[/latex] | −0.4030 |
[latex]\text{Fe}^{2+}(aq)\;+\;2\text{e}^{-}\;{\longrightarrow}\;\text{Fe}(s)[/latex] | −0.447 |
[latex]\text{Cr}^{3+}(aq)\;+\;3\text{e}^{-}\;{\longrightarrow}\;\text{Cr}(s)[/latex] | −0.744 |
[latex]\text{Zn}^{2+}(aq)\;+\;2\text{e}^{-}\;{\longrightarrow}\;\text{Zn}(s)[/latex] | −0.7618 |
[latex]2\text{H}_2\text{O}(l)\;+\;2\text{e}^−\longrightarrow\;\text{H}_2(g)\;+\;2\text{OH}^−(aq)[/latex] | −0.8277 |
[latex]\text{Mn}^{2+}(aq)\;+\;2\text{e}^{-}\;{\longrightarrow}\;\text{Mn}(s)[/latex] | −1.185 |
[latex]\text{Zn(OH)}_2(s)\;+\;2\text{e}^{-}\;{\longrightarrow}\;\text{Zn}(s)\;+\;2\text{OH}^{-}(aq)[/latex] | −1.245 |
[latex]\text{Al}^{3+}(aq)\;+\;3\text{e}^{-}\;{\longrightarrow}\;\text{Al}(s)[/latex] | −1.662 |
[latex]\text{Mg}^{2+}(aq)\;+\;2\text{e}^{-}\;{\longrightarrow}\;\text{Mg}(s)[/latex] | −2.372 |
[latex]\text{Na}^{+}(aq)\;+\;\text{e}^{-}\;{\longrightarrow}\;\text{Na}(s)[/latex] | −2.71 |
[latex]\text{Ca}^{2+}(aq)\;+\;2\text{e}^{-}\;{\longrightarrow}\;\text{Ca}(s)[/latex] | −2.868 |
[latex]\text{Ba}^{2+}(aq)\;+\;2\text{e}^{-}\;{\longrightarrow}\;\text{Ba}(s)[/latex] | −2.912 |
[latex]\text{K}^{+}(aq)\;+\;\text{e}^{-}\;{\longrightarrow}\;\text{K}(s)[/latex] | −2.931 |
[latex]\text{Li}^{+}(aq)\;+\;\text{e}^{-}\;{\longrightarrow}\;\text{Li}(s)[/latex] | −3.04 |
Table 1 Selected Standard Reduction Potentials at 25 °C. Source: OpenStax Chemistry 2e |
It is interesting to note that water, Sn2+, and many other transition metal ions in Table 1 appear as both an oxidizing agent and a reducing agent, depending on the context. We know that water does not react spontaneously with itself and the reason is the reduction potential of water as an oxidizing agent is lower than the reduction potential of water as a reducing agent.
Example 1
Use Table 1 to predict whether the following combinations will result in a redox reaction. If a reaction can occur, identify the reducing agent, reduction half-reaction, oxidizing agent, oxidation half-reaction, and the overall redox reaction.
(a) O2(g), H+(aq), Cu(s)
(b) F2(g), H2O(l)
(c) Na(s), H2O(l)
(d) Na+(aq), Cl−(aq)
(e) PbO2(s), SO42−(aq), H+(aq), Pb(s), PbSO4(s)
(f) Sn2+(aq), H2(g)
SOLUTION
(a) Species: O2(g), H+(aq), Cu(s), H2O(l). Water is not explicitly listed but is the solvent in the aqueous solution.
Oxidizing agents: O2(g) + H+(aq) (E° = +1.229 V), H+(aq) (E° = 0 V), H2O(l) (E° = −0.8277 V)
The strongest oxidizing agent has the highest E° and is the combination O2(g) + H+(aq).
Reducing agents: Cu(s) (E° = +0.337 V), H2O(l) (E° = +1.229 V)
The strongest reducing agent has the lowest E° and is Cu(s).
[latex]\begin{align*} &\text{reduction:}&\;\text{O}_2(g)\;+\;4\text{H}^{+}(aq)\;+\;4\text{e}^{-}\;&{\longrightarrow}\;2\text{H}_2\text{O}(l)) \\ &\text{oxidation:}&2\times \{\;\text{Cu}(s)\; &{\longrightarrow}\;\text{Cu}^{2+}(aq)\;+\;2\text{e}^{-} \} \\ \hline &\text{overall:}& \;\text{O}_2(g)\;+\;4\text{H}^{+}(aq)\;+\;2\text{Cu}(s)\;&{\longrightarrow}\;2\text{H}_2\text{O}(l)\;+\;2\text{Cu}^{2+}(aq) \end{align*}[/latex]
(b) Species: F2(g), H2O(l)
Oxidizing agents: F2(g) (E° = +2.866 V), H2O(l) (E° = −0.8277 V)
The strongest oxidizing agent has the highest E° and is F2(g).
Reducing agent: H2O(l) (E° = +1.229 V)
The only reducing agent is H2O(l).
[latex]\begin{align*} &\text{reduction:}&2\times\left\{\text{F}_2(g)\;+\;2\text{e}^{-}\;\right.&{\longrightarrow}\;\left.2\text{F}^-(aq)\right\} \\ &\text{oxidation:}&\;2\text{H}_2\text{O}(l) \;&{\longrightarrow}\;\text{O}_2(g) \;+\;4\text{H}^{+}(aq)\;+\;4\text{e}^{-} \\ \hline &\text{overall:}&\;2\text{F}_2(g)\;+\; 2\text{H}_2\text{O}(l) \;&{\longrightarrow}\;4\text{F}^-(aq) \;+\;\text{O}_2(g) \;+\;4\text{H}^{+}(aq) \end{align*}[/latex]
(c) Species: Na(s), H2O(l)
Oxidizing agent: H2O(l) (E° = −0.8277 V)
The only oxidizing agent is H2O(l).
Reducing agents: Na(s) (E° = −2.71 V), H2O(l) (E° = +1.229 V)
The strongest reducing agent has the lowest E° and is Na(s).
[latex]\begin{align*} &\text{reduction:}&2\text{H}_2\text{O}(l)\;+\;2\text{e}^{-}\;&{\longrightarrow}\;\text{H}_2(g) \;+\;2\text{OH}^{-}(aq) \\ &\text{oxidation:}&2\times\{\;\text{Na}(s) \;&{\longrightarrow}\;\text{Na}^{+}(aq)\;+\;\text{e}^{-}\} \\ \hline &\text{overall:}&\;2\text{H}_2\text{O}(l)\;+\;2\text{Na}(s)\;&{\longrightarrow}\;\text{H}_2(g) \;+\;2\text{OH}^{-}(aq)\;+ \;2\text{Na}^{+}(aq)\end{align*}[/latex]
(d) Species: Na+(aq), Cl−(aq), H2O(l). Water is not explicitly listed but is the solvent in the aqueous solution.
Oxidizing agents: Na+(aq) (E° = −2.71 V), H2O(l) (E° = −0.8277 V)
The strongest oxidizing agent has the highest E° and is H2O(l).
Reducing agents: Cl−(aq) (E° = +1.35827), H2O(l) (E° = +1.229 V)
The strongest reducing agent has the lowest E° and is H2O(l).
There is no redox reaction because the strongest oxidizing agent H2O(l) (E° = −0.8277 V) is lower than the strongest reducing agent H2O(l) (E° = +1.229 V) on the E° list.
(e) Species: PbO2(s), SO42−(aq), H+(aq), Pb(s), PbSO4(s), H2O(l). Water is not explicitly listed but is the solvent in the aqueous solution.
Oxidizing agents: PbO2(s) + SO42−(aq) + H+(aq) (E° = +1.69 V), H2O(l) (E° = −0.8277 V)
The strongest oxidizing agent has the highest E° and is the combination PbO2(s) + SO42−(aq) + H+(aq).
Reducing agents: Pb(s) + SO42−(aq) (E° = −0.3505 V), Pb(s) (E° = −0.126), H2O(l) (E° = +1.229 V), PbSO4(s) + H2O(l) (E° = −1.69 V)
The strongest reducing agent has the lowest E° and is the combination Pb(s) + SO42−(aq).
[latex]\begin{align*} &\text{reduction:}& \;\text{PbO}_2(s) \;+\;\text{SO}_4^{2-}(aq) \;+\;4\text{H}^{+}(aq)\;+\;2\text{e}^{-}\;&{\longrightarrow}\;\text{PbSO}_4(s) \;+\;2\text{H}_2\text{O}(l) \\ &\text{oxidation:}&\;\text{Pb}(s) \;+\;\text{SO}_4^{2-}(aq) \;&{\longrightarrow}\;\text{PbSO}4 (s)\;+\;2\text{e}^{-} \\ \hline &\text{overall:}&\; \text{PbO}_2(s) \;+\;2\text{SO}_4^{2-}(aq) \;+\;4\text{H}^{+}(aq)\;+\;\text{Pb}(s)\;&{\longrightarrow}\;2\text{PbSO}_4(s)\;+\; 2\text{H}_2\text{O} (l) \end{align*}[/latex]
This reaction takes place in a lead acid car battery!
(f) Species: Sn2+(aq), H2(g), H2O(l). Water is not explicitly listed but is the solvent in the aqueous solution.
Oxidizing agents: Sn2+(aq) (E° = −0.1262), H2O(l) (E° = −0.8277 V)
The strongest oxidizing agent has the highest E° and is Sn2+(aq).
Reducing agents: Sn2+(aq) (E° = +0.151), H2O(l) (E° = +1.229 V), H2(g) (E° = 0 V)
The strongest reducing agent has the lowest E° and is H2(g).
There is no redox reaction because the strongest oxidizing agent Sn2+(aq) (E° = −0.1262) is lower than the strongest reducing agent H2(g) (E° = 0 V) on the E° list.
The standard reduction potentials in Table 1 are measured by observing the reduction and oxidation reactions in half-cells, just as we analyzed reduction and oxidation as separate half-reactions. The reduction half-reaction occurs in one half-cell, and the oxidation half-reaction in the other half-cell. We can observe changes in electrode mass, solution colour, and/or pH to distinguish between whether the reaction in the half-cell is reduction or oxidation. A wire carries electrons from the oxidation half-cell to the reduction half-cell. The two half-cells together form a complete electrochemical cell corresponding to the overall redox reaction. The potential difference or voltage between the two half-cells, measured in volts (V) by a voltmeter is the difference in electrical potential between the reduction and oxidation half-reactions.
The standard reduction potential of the half-reaction
[latex]2\text{H}^+(aq)+2\text{e}^-\longrightarrow H_2(g)[/latex] [latex]E^{\circ}=0\text{ V}[/latex]
is the electrical potential of the standard hydrogen electrode (SHE) that is set as the reference point for measuring reduction potentials. “Standard” refers to a concentration of 1 M for solute species and a partial pressure of 1 atm or 1 bar for gases. The SHE half-cell consists of a platinum electrode suspended in an electrolyte solution containing 1 M H+(aq) in which hydrogen gas at 1 atm is bubbled through the solution, Figure 1. The electrode is an electrically conductive solid material that serves as the surface for redox reactions. The electrolyte is the electrically conductive solution in a half-cell.
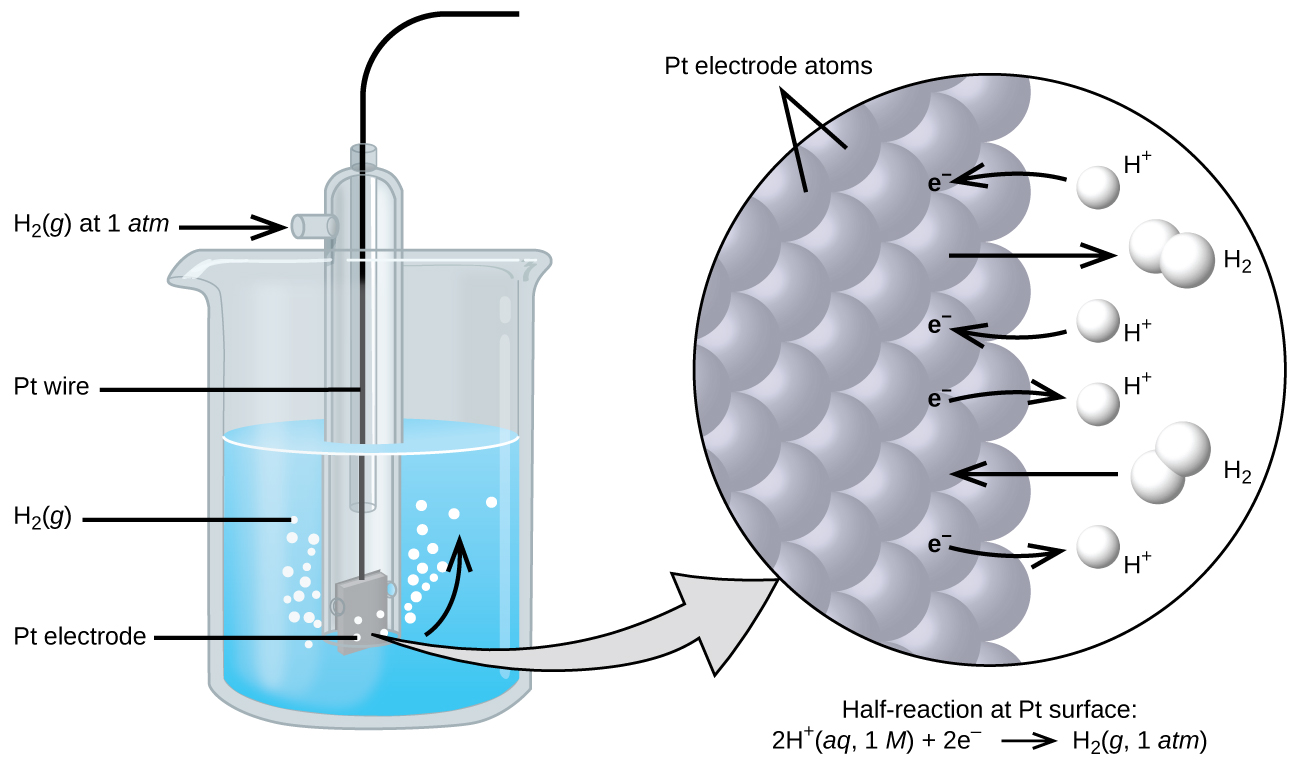
To measure the standard reduction potential of another species, such as copper, a Cu(s) electrode is immersed in a solution of 1 M Cu2+ and connected by a wire to the SHE, Figure 2. The hydrogen and copper half-cells are the compartments in which the oxidation and reduction half-reactions take place, respectively. We can identify the reduction half-reaction as Cu2+ + 2 e− → Cu by observing that the Cu(s) electrode increases in mass as Cu2+ is reduced to metallic copper on the electrode. Also, because Cu2+ in solution appears blue, the decrease in Cu2+ causes the blue colour to fade. We can identify the oxidation half-reaction as H2 → 2 H+ + 2 e− because the solution of the hydrogen half-cell becomes more acidic as hydrogen gas is oxidized to H+.
[latex]\begin{align*} &\text{reduction:}&\;\text{Cu}^{2+}(aq)\;+\;2\text{e}^{-}\;&{\longrightarrow}\;\text{Cu}(s) \\ &\text{oxidation:}&\;\text{H}_2(g) \;&{\longrightarrow}\;2\text{H}^{+}(aq)\;+\;2\text{e}^{-} \\ \hline &\text{overall:}&\;\text{Cu}^{2+}(aq)\;+\;\text{H}_2(g)\;&{\longrightarrow}\;\text{Cu}(s)\;+\;2\text{H}^+(aq) \end{align*}[/latex]
There is a measurable potential difference in the wire that carries electrons from the platinum SHE to the copper electrode. Because the SHE reduction potential is set as the reference point zero, any potential difference measured relative to the SHE will be the standard reduction potential of the copper reduction half-reaction E° = +0.337 V. The positive potential difference of +0.337 V means that Cu2+ has a greater tendency to accept electrons compared to H+.
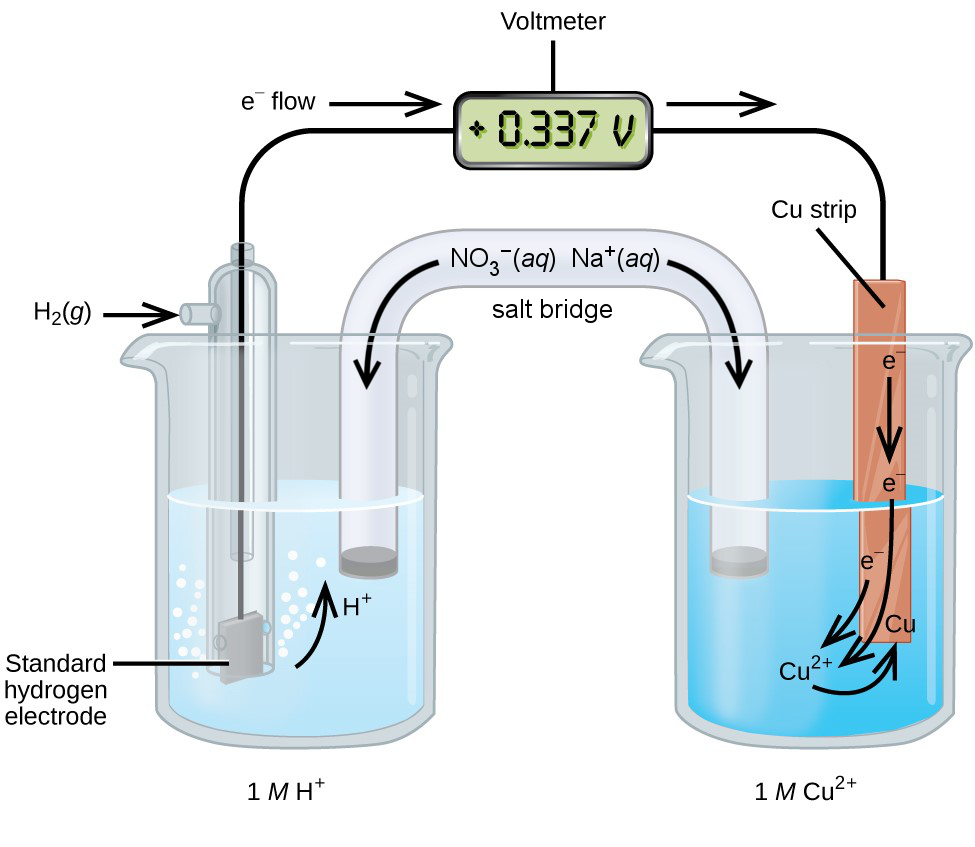
The hydrogen-copper redox reaction changes the ionic composition of the half-cells. To restore electrical balance, a salt bridge containing an inert electrolyte such as NaNO3 allows the cation Na+ to migrate to the copper half-cell and the anion NO3− to migrate to the hydrogen half-cell. The consumption of Cu2+ leads to a decrease in positive charge in the electrolyte of the copper half-cell that is balanced by the influx of Na+. The production of H+ increases the positive charge in the electrolyte of the hydrogen half-cell and is balanced by the counterion NO3−. The ions Na+ and NO3− are inert because they are not oxidized or reduced by the hydrogen-copper redox reaction.
The copper half-cell can be replaced by another half-cell, such as a standard silver half-cell, to measure the standard reduction potential, Figure 3. We can tell that Ag+ is reduced to elemental Ag(s) because the electrode mass increases. The hydrogen gas is oxidized as the solution of the hydrogen half-cell becomes more acidic with increasing H+.
[latex]\begin{align*} &\text{reduction:}&\;2\times \{\text{Ag}^{+}(aq)\;+\;\text{e}^{-}\;&{\longrightarrow}\;\text{Ag}(s)\} \\&\text{oxidation:}&\;\text{H}_2(g) \;&{\longrightarrow}\;2\text{H}^{+}(aq)\;+\;2\text{e}^{-} \\ \hline &\text{overall:}&\;2\text{Ag}^{+}(aq)\;+\;\text{H}_2(g)\;&{\longrightarrow}\;2\text{Ag}(s)\;+\;2\text{H}^+(aq) \end{align*}[/latex]
Electrons flow from the SHE to the silver electrode. The potential difference of +0.80 V between the SHE and the standard Ag/Ag+ half-cell is the standard reduction potential for the half-reaction Ag+ + e− → Ag. Ag+ (E° = +0.80 V) has a more positive E° meaning greater desire for electrons than H+ (E° = 0 V) and also Cu2+ (E° = +0.337 V).
The reduction of Ag+ to elemental Ag decreases the positive charge in the solution of the silver half-cell, and the production of H+ from H2 increases the positive charge in the solution of the hydrogen half-cell. The inert ions in the salt bridge restore electrical balance in the half-cells. Cationic Na+ migrates to the silver half-cell, and anionic NO3− to the hydrogen half-cell.
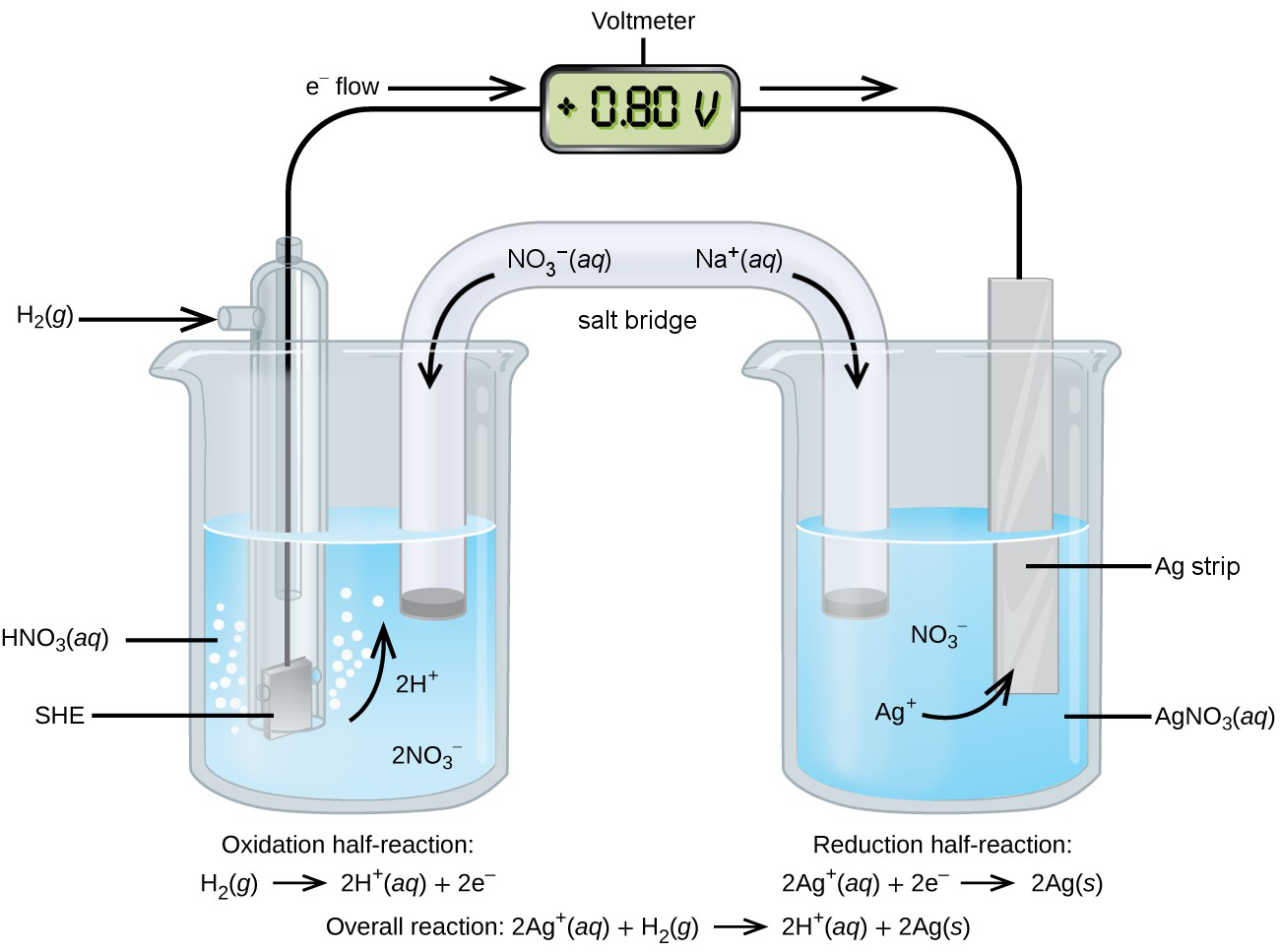
Unlike the copper and silver half-cells, connecting a zinc half-cell to the SHE results in oxidation of the zinc electrode. The mass of zinc decreases as metallic zinc is oxidized to Zn2+. In the hydrogen half-cell, the solution becomes more basic as H+ is reduced to bubbles of hydrogen gas that forms on the platinum electrode, Figure 4.
[latex]\begin{align*} &\text{reduction:}&\;2\text{H}^{+}(aq)\;+\;2\text{e}^{-}\;&{\longrightarrow}\;\text{H}_{2}(g) \\ &\text{oxidation:}&\;\text{Zn}(s) \;&{\longrightarrow}\;\text{Zn}^{2+}(aq)\;+\;2\text{e}^{-} \\ \hline &\text{overall:}&\;2\text{H}^{+}(aq)\;+\;\text{Zn}(s)\;&{\longrightarrow}\;\text{H}_{2}(g)\;+\;\text{Zn}^{2+}(aq) \end{align*}[/latex]
The zinc electrode gives electrons to the SHE. The negative potential difference of −0.76 V between the zinc electrode and the SHE means that Zn2+ has a lower tendency to be reduced than H+. The standard reduction potential of the Zn2+ + 2 e− → Zn half-reaction is E° = −0.76 V.
The reduction of H+ decreases the positive charge in the solution of the hydrogen half-cell, and the oxidation of Zn to Zn2+ increases the positive charge in the solution of the zinc half-cell. From the salt bridge, the cation Na+ migrates to the hydrogen half-cell and the anion NO3− migrates to the zinc half-cell to restore electrical balance.
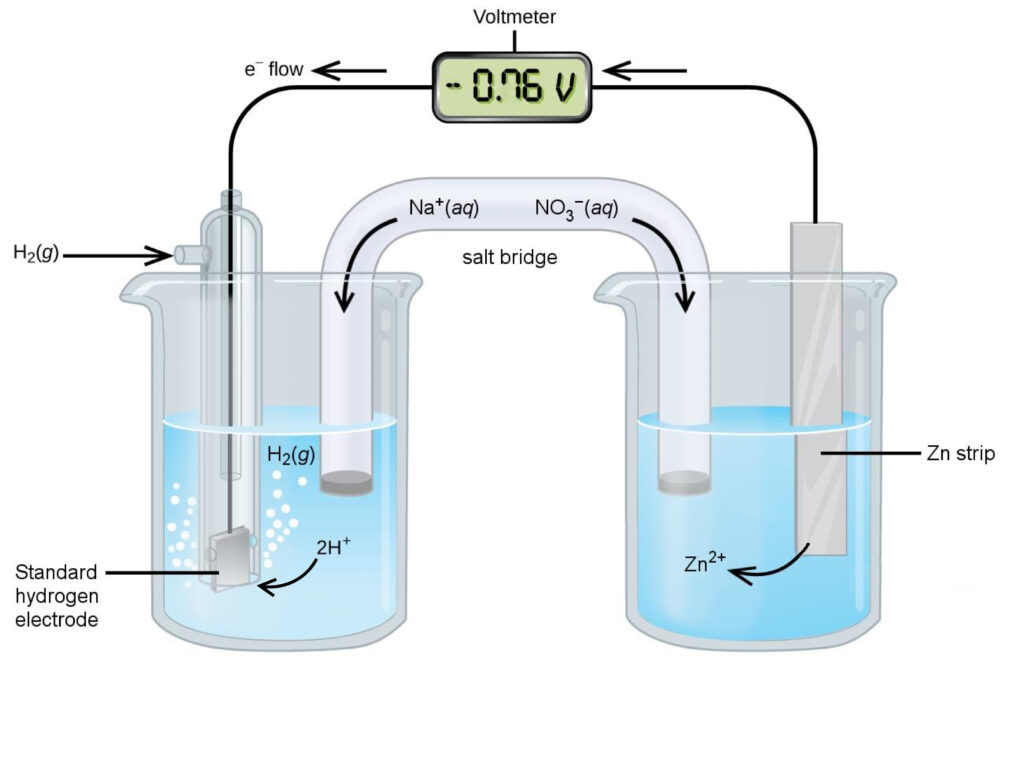
The SHE is rather dangerous, because hydrogen gas is explosive and acid is corrosive, and rarely used in the laboratory. Its main significance is that it established the zero for standard reduction potentials. Once determined, standard reduction potentials can be used to determine the spontaneity and standard cell potential, E°cell, for any electrochemical cell.
Inert Electrodes
The electrode is a surface for redox reactions and has to be an electrically conductive solid, which is why the electrode in the hydrogen half-cell is platinum metal. The other components in the hydrogen half-cell are aqueous hydrogen ions, counterions, hydrogen gas, and water and none are solids. Platinum is a conductive metal that is inert, meaning that platinum does not react with H+ or H2 and thus does not interfere with the hydrogen half-reaction. Gold is another common inert electrode. Platinum and gold are quite expensive. A more economical choice for an inert electrode is a carbon rod.
The electrodes used in the copper and silver half-cells are the reduced forms of the oxidizing agents Cu2+ and Ag+. We may also substitute the copper and silver electrodes with another electrically conductive solid that is inert to Cu2+ and Ag+. However, as Zn(s) is the reducing agent in the zinc half-cell, it is necessary to include the metal zinc electrode.
Example 2
Describe a method by which the standard reduction potential of chlorine gas can be measured using half-cells.
SOLUTION
Standard reduction potentials measured relative to the SHE require the standard hydrogen half-cell that consists of a platinum electrode in a solution of 1 M H+(aq) and 1 atm H2(g). The 1 M H+(aq) solution can be 1 M HNO3(aq). The chlorine half-cell has 1 atm Cl2(g) bubbled in a solution of 1 M Cl−(aq). The 1 M Cl−(aq) solution can be 1 M NaCl(aq). An inert electrode is needed for the chlorine half-cell. Platinum is not an appropriate electrode because the reduction potential of the half-reaction Pt2+(aq) + 2 e− ⟶ Pt(s) (E° = +1.20 V) is below that of chlorine, Cl2(g) + 2 e− ⟶ 2 Cl−(aq) (E° = +1.35827 V). The chlorine gas will react with platinum. If platinum is used as the electrode in the chlorine half-cell, the oxidation of the electrode by chlorine will indicate that another material is needed. Gold is a good choice as an inert electrode because the reduction potential of Au3+(aq) + 3 e− ⟶ Au(s) (E° = +1.498 V) is above that of chlorine. Chlorine will not react with gold. The voltmeter reading between the hydrogen and chlorine half-cells is the standard reduction potential of chlorine E° = +1.35827 V.
The hydrogen and chlorine reactions are
[latex]\begin{align*} &\text{reduction:}&\;\text{Cl}_2 (g)\;+\;2\text{e}^{-}\;&{\longrightarrow}\;2\text{Cl}^{-}(aq) \\ &\text{oxidation:}&\;\text{H}_2(g) \;&{\longrightarrow}\;2\text{H}^{+}(aq)\;+\;2\text{e}^{-} \\ \hline &\text{overall:}&\; \text{Cl}_2 (g)\;+\;\text{H}_2(g)\;&{\longrightarrow}\;2\text{Cl}^{-} (aq)\;+\;2\text{H}^{+}(aq) \end{align*}[/latex]
In the hydrogen half-cell, the pH decreases as hydrogen gas is oxidized to H+. Chlorine gas is reduced to Cl− in the chlorine half-cell. An inert electrolyte solution like NaNO3 can be used in the salt bridge. To maintain electrical balance, NO3−(aq) will migrate to the hydrogen half-cell and Na+(aq) will migrate to the chlorine half-cell.
Chemistry in Real Life
Redox Reactions of Biological Molecules
We are familiar with the exchange of electrons between elements and ions in aqueous solution. Organic molecules, including metabolites, also participate in redox reactions. In organic redox reactions, reduction often involves the carbon chain gaining hydrogen atom(s) and/or losing oxygen atom(s). The reverse is the case for oxidation, where the carbon chain loses hydrogen atom(s) and/or gains oxygen atom(s).
Vitamin C, also known as ascorbic acid, maintains skin resiliency by crosslinking the collagen protein of skin. A chronic lack of vitamin C causes scurvy. Moreover, vitamin C reduces free radicals that would otherwise oxidize and damage cellular components. These free radicals can be by-products of cellular metabolism. Vitamin C is oxidized as follows,
The symbol “[O]” means a generic oxidation reaction without specifying the oxidizing agent, as there are multiple metabolites that can oxidize vitamin C. Notice that vitamin C loses the red hydrogen atoms of the hydroxyl (‒OH) groups to form carbonyl (‒C═O) groups.
Vitamin C may be associated with lemons and oranges, but citrus did not originate from North America. Instead, spruce needles have been a source of vitamin C for West coast Indigenous peoples, who make tea from spruce needles.
Be careful about eating from trees without being sure about the species. Some spruce species are not good for eating, such as the trees with blue-green needles that are silvery grey under the branches.
Data Table
Standard reduction potentials for select substances (Source: OpenStax Chemistry 2e). The table is listed in alphabetical order of the chemical symbol and not in order of the reduction potentials.
Optional Activity
Explore this simulation on redox chemistry. There are four activities in total. In each activity, a solid metal is dipped into a solution. Sometimes, there is evidence of reaction, such as the plating of another metal on the first metal or the evolution of gas. Other times, no reaction occurs. Based on the table of reduction potentials, can you predict which of the metals will react with which solution and what are the products of reaction?
We measure the standard reduction potential of an unknown compound relative to that of the standard hydrogen electrode. If the bubbles of hydrogen gas appear on the hydrogen electrode and the electrolyte pH increases in the hydrogen half-cell, then the unknown compound:
A) is oxidized and has a more negative reduction potential than that of hydrogen.
B) is reduced and has a more negative reduction potential than that of hydrogen.
C) is oxidized and has a more positive reduction potential than that of hydrogen.
D) is reduced and has a more positive reduction potential than that of hydrogen.
Click to see answer
A) is oxidized and has a more negative reduction potential than that of hydrogen.